The Importance of Visual Inspection in Injection of Parenteral Drug Products
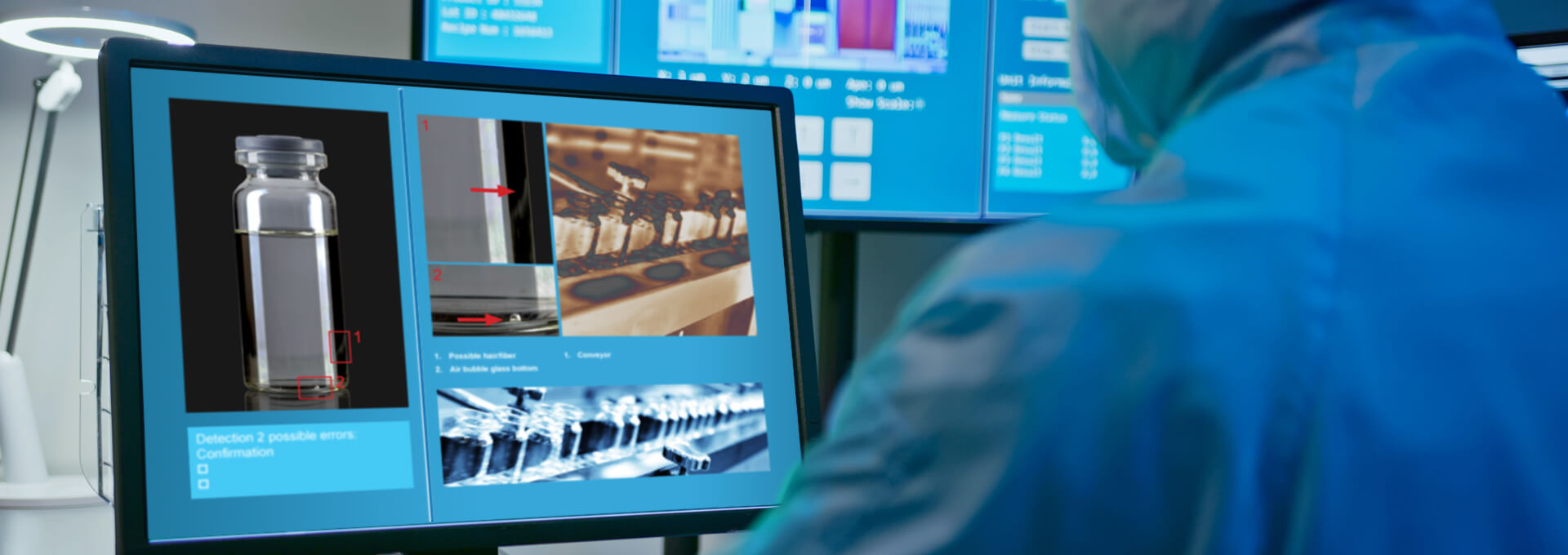
Regulatory Landscape
The United States (U.S) Food and Drug Administration’s (FDA) draft guidance on visual inspection (1) states that visible particulate control should be developed and implemented in a holistic approach that incorporates product development, manufacturing control, visual inspection techniques and particulate identification to assess and prevent the risk of visible particle contamination. According to the guidance document, the clinical manifestation of adverse events caused by particulate contamination depends on the route of administration, patient population and nature, size and shape of particulates, and chemical reactivity to certain cells or tissues.
While the call for injectable products to be free of any visible particle contamination has been described in the regulations, the varied probability of detecting particles during nondestructive inspection has also been officially recognized. This recognition is expressed in one sentence: “Injectable solutions, including solutions constituted from sterile solids intended for parenteral use, should be essentially free from particles that can be observed on visual inspection” (2). Regulatory monographs in Europe and the U.S. require drug products for parenteral administration to be "practically free" or "essentially free" of visible particles, respectively. Both terms have been used interchangeably and acknowledge the probabilistic nature of visual particle inspection.
Requirements Based on Risk
The FDA draft guidance document discusses implementing a risk-based approach to visible particulates. Meeting the acceptance criteria defined in United States Pharmacopeia (USP) General Chapter <790> alone is not sufficient in achieving compliance with overall current good manufacturing practices requirements. The patient risk to particulates varies based on the route of administration, patient population and the type of particulates. These factors must be considered when developing a particulate control strategy and establishing acceptance criteria.
Unlike conventional small-molecule drugs, many of which can be formulated effectively with excipients to avoid degradation in the stomach and exhibit reasonably efficient uptake through the gastrointestinal track, biological drugs may exhibit lower stability and a greater sensitivity to enzymatic degradation, making oral delivery problematic. As biologic-based therapeutic agents are developed, and the benefit of localized delivery is acknowledged, the potential routes of administration have increased in variety to address the efficacy and safety requirements. Route of administration is one of the primary contributors to patient risk in relation to particulate contamination. Therefore, it is essential to understand the physiological outcomes of particulate contamination according to anatomy and tissue type. The majority of biologics are currently administered through subcutaneous or intramuscular injection or via intravenous (IV) infusion. In addition, several alternative routes of administration are indicated for commercialized biologic drug products and are being intensively investigated at the preclinical and clinical stages. Visual inspection strategies should be developed commensurate with patient risk.
Probabilistic Nature of Visual Inspection and Needle Gauges Used in Administration
USP <790> establishes the precedent that each finished unit of an injectable product will be inspected as part of the routine manufacturing process. There are three strategies for performing 100% visual inspection: manual, semi-automated and automated. Manual visual inspection involves examining product units with the unaided eye (except for optical correction required to establish normal vision) by inspectors. In contrast, semi-automated inspection may implement a conveyor or transport system to present the units to the inspectors. Automated visual inspection implements image analyses or light obscuration instead of engaging inspectors. Moreover, manual, semi-automated and automated inspection methods may be combined, depending on the specific product and the visual inspection requirements determined through risk assessments.
Manual visual inspection often exhibits variability due to many factors, such as container design, particle transparency, particle aspect ratio, and training and inspector fatigue, which impacts the results. The probability of detecting particles based on particle size has been examined in multiple studies. Melchore et al. summarize these studies in which the probability of detection ranges from 0% for a particle size of 50 µm to 100% at 200 µm (3).
On the other hand, needle gauge could determine the largest particle size introduced into the body. Table 1 lists the commonly used needle gauges and the corresponding inner-lumen diameters (4):
Needle Gauge | Inner Diameter (mm) |
---|---|
16 | 1,190 |
17 | 1,067 |
18 | 838 |
19 | 686 |
20 | 603 |
21 | 514 |
22 | 413 |
23 | 337 |
24 | 311 |
25 | 260 |
26 | 260 |
27 | 210 |
28 | 184 |
29 | 184 |
30 | 159 |
31 | 133 |
32 | 108 |
33 | 108 |
34 | 51 |
The smallest inner-diameter needles are 34-gauge used for superficial injections (insulin) and puncture of the epidermis, whereas intramuscular injections can be performed with 18-gauge needles. Inner needle diameters cannot exclude particulates smaller than their inner diameter that have not been detected by visual inspection unless additional filters are applied during the injection process. However, filters are not universal across healthcare systems and are not generally applicable to “at-home” injections.
Route of Administration and Adverse Events
The Visual inspection strategy is intended to reduce the adverse events associated with particulate contamination of parenteral injections. The most common routes of administration and the severities of particulate contamination outcomes are discussed in the article.
Vascular Injections
When particulate matter is administered into a vessel, the matter will travel in the direction of blood flow until the diameter of the vessel is smaller than the particulate, and the matter becomes lodged in the vessel. This lodged particulate will ultimately restrict blood flow to or from the tissues distal to the blockage. Particulates in intravascular (IV) injections generally can cause more adverse events than those in subcutaneous or intramuscular injections. The clinical impact of an occluded vessel depends on many variables, including the size of the vessel occluded and the number of vessels supplying a particular organ (collateral blood flow) (5). In many instances, there may be no significant, immediate clinical impact as some tissues or organs have extensive blood vasculature, so numerous vessels may feed the same tissue. Therefore, if any number of small vessels become occluded, the blood flow to the tissue may not be significantly impacted, and thus, the patient may have no symptoms.
The size of potential particles entering the vascular system through IV can be estimated based on IV needle diameters. The World Health Organization (WHO) released guidelines regarding best practices for injection and provided specific guidance for choosing a needle for IV injection based on the need for the needle to fit into the most prominent vein with the least discomfort, as shown below in Table 2 (6).
Table 2 WHO Best Practices for Injections and Related Procedures Toolkit (6)Needle Gauge |
Patient Population |
||
---|---|---|---|
Adult | Pediatric, Elderly, Small Veins | Neonatal | |
16/18 | No (unless blood donation) | No | No |
19/20 | No | No | No |
21 | Yes | No | No |
22 | Yes | Yes | No |
23 | Yes | Yes | Yes |
If all injections were performed according to this guidance, the largest particle that could be injected into an adult would be approximately 514 µm in diameter; in pediatrics and elderly, it would be 413 µm in diameter, and in neonatal patients, it would be 327 µm in diameter.
Intra-Arterial Injections, Including Intracoronary
Arteries carry oxygenated blood from the heart to the rest of the body. Because arteries decrease in size with the direction of blood flow, particulate matter injected intra-arterially will travel distally until it becomes lodged in a vessel due to the particle’s size. Thus, the inadvertent administration of intra-arterially injected particles that are too large to pass through arterioles and capillaries may cause occlusions that could affect blood flow to tissues downstream of the injection site, potentially causing tissue ischemia and infarction. The physiological effects of any such occlusion will depend upon the size of the particle and the collateral circulation available to the affected area. Ironically, smaller particles capable of blocking terminal arterial vessels—and causing infarctions—may be more detrimental than larger particles capable of arteriole occlusion due to the reduced collateral blood supply available to the affected tissue.
One of the most critical locations for intra-arterial administration is the injection into the arteries that bring blood to the brain and spinal cord. Vascular embolic events from intra-arterial injection of particulate corticosteroids have been found to account for serious complications, including spinal cord infarction, paraplegia and death (7). Arterial injection of particulate methylprednisolone has been shown to cause severe neurological consequences, such as unconsciousness and cerebral hemorrhage. Benzon et al. looked at the size of particles in different dilutions of steroid injections, and they noted that some formulations have particles as large as 1,000 µm. These particles are large enough to completely occlude the ascending cervical and deep cervical arteries and to partially occlude the vertebral artery if accidentally injected into an artery (8). While smaller particles (10-50 μm) may not be able to occlude the arteries, these smaller particles can occlude the arterioles and capillaries, causing tissue damage. It is, therefore, possible that any particulate steroid can occlude the distal portions of the arteries.
Intravenous Injections, Including Intravenous Bolus and Intravenous Drip
Veins carry deoxygenated blood from the tissues back to the right side of the heart, which pumps it to the lungs for reoxygenation. Because the size of veins increases in the direction of blood flow, most particles injected intravenously will travel through the venous system to the heart on their way to the lungs via the pulmonary artery. If the particles are small enough to fit through the lumen of the IV catheter, they would be expected to reach the heart and then the lungs. The particles would likely lodge in the pulmonary vasculature and form granulomas or cause potential downstream ischemia or tissue injury. Given the large availability of collateral blood supply in the lungs, which increases distally, the clinical effects for the average patient population are thought to be minimal. Langille et al. reported that the average diameter for pulmonary vasculature is approximately 6-8 µm (9). Based on this data, particles larger than 6-8 µm will likely remain embedded in pulmonary capillaries, while smaller particles can pass through the lungs and become lodged in other organs, such as the liver and spleen.
Further information regarding the harm of particulate matter injected intravenously can be obtained from case reports about IV drug abusers and complications in patients dependent on total parenteral nutrition (TPN). In the cases of IV drug abusers, a powder or pulverized tablet is suspended in a liquid vehicle and then variably filtered through cotton, which could introduce both drug substance particulate matter and cotton fibers intravascularly. These patients may develop dyspnea and show reduced pulmonary function testing. This highlights that a localized pulmonary inflammatory response may be more likely to result from particles than pulmonary embolism or clinically symptomatic tissue damage. TPN associated with inherent particles (calcium and phosphate) administered to pigs has been shown to cause respiratory distress and sudden death with amorphous calcium-phosphate precipitant debris (10).
Ophthalmologic Injections, Including Conjunctival, Intracorneal, Intraocular, Intravitreal, Retrobulbar and Subconjunctival
The eye contains several distinct spaces in which medications can be administered. Injection of particulate matter into the contained space of the eye can cause direct harm to the intraocular structures or can travel into aqueous ducts and cause blockages. If particles are injected into a contained space (intraocularly), there may be a higher risk for particles to cause inflammation or serve as a nidus for infection. The eye is one of a few areas of the body with limited exposure to the body’s immune system, a phenomenon known as immune privilege. The eye limits its inflammatory immune response, so swelling and other tissue changes do not harm vision. If infectious material is introduced into this privileged space, due to this diminished immune response, the infection and tissue damage can be very serious to the point of permanent blindness.
Age-related macular degeneration, diabetic retinopathy and retinal vein occlusion are the most common conditions treated with intravitreal injections. The frequency of intravitreal injections has significantly increased since the introduction of anti-vascular endothelial growth factor medications. Given that the vitreous is a small, contained space and that neovascular retinal diseases typically require ongoing treatment, the risks associated with injecting particulate matter (including silicone oil) into the vitreous may be cumulative. In addition, particles may be associated with complications, such as floaters, sustained increase in intraocular pressure and endophthalmitis (11, 12).
Soft Tissue Injection-Intramuscular and Subcutaneous
Needle gauges used for intramuscular injections can be 19-25 gauge. This means that the sizes of injected particulate matter can range from a maximum of 260 µm to 686 µm in diameter. Subcutaneous injections use needle gauges of 25 or greater. Immunologically inert particles, such as glass or cellulosic fibers, delivered via intramuscular and subcutaneous routes have received little attention regarding their potential for causing adverse events. Because the delivered volumes (and the overall particle load) are relatively small, the risk of a systemic reaction is low and the ability of these particles to migrate far from the injection site is negligible. The particles would be too large to be phagocytosed by tissue macrophages. The most likely outcome from particulate matter administered intramuscularly is minor irritation or development of a granuloma. For intramuscular injections, Greenblatt and Allen looked at 26,294 hospitalized medical patients, 46% of whom received at least one intramuscular injection, and found that clinically apparent local complications are uncommonly (less than 0.4%) associated with intramuscular injections (13).
CNS Intracerebral Injections, Intracisternal, Intrameningeal, Intraspinal and Subarachnoid
Whenever a drug is injected into a contained space, such as for intrathecal (spinal canal/subarachnoid) use, there may be more risk for inflammation from particles, or a particle may serve as a nidus for infection, causing harm. Brierley et al. established that there is free passage of particulate matter from sub-arachnoid to sub-dural space and then towards the peripheral nerve (14). Thus, any particulate matter injected into the intrathecal space would have the ability to travel cephalically or caudally, potentially affecting neural tissue in the spinal cord and the brain. There is limited data available, but the presence of particles in solutions for intrathecal use has been reported from the use of drugs within glass ampoules, potentially causing symptoms from nerve root involvement or worsening lumbar back pain.
Particulate matter injected intrathecally could also cause harm due to direct contact with the spinal canal and nerves. Subdural and intrathecal spread or diffusion of the injected mixture of corticosteroids, anesthetic, and contrast dye could result in cauda equina and conus medullaris syndromes, arachnoiditis, meningitis, and temporary respiratory depression. This contact could also cause permanent loss of movement, sensation and coordination loss, depending on the location affected.
There is also a significant risk of a large particulate causing an obstruction to the aqueduct of Sylvius, which is the narrowest point in the cerebral spinal fluid (CSF) pathway. The cerebral aqueduct is a narrow 15 mm long and 1-2 mm wide conduit that allows for CSF to flow between the third and fourth ventricles. Obstruction of the aqueduct of Sylvius would cause obstructive hydrocephalus. In addition, particulates in the ventricular space can also lead to obstructive hydrocephalus by obstructing the outflow of CSF from the ventricular system. This outflow obstruction causes increased intracranial pressure, potentially resulting in brain herniation and death.
Conclusions
Particulate contamination in parenteral injections is a patient risk factor that needs to be understood, analyzed and controlled. The route of administration and the potential migration of particulates determine the severity of the outcome of the adverse events caused by particulates. Visual inspection, as part of a holistic approach to control product contamination, plays a significant role in diminishing patient risk.
Want To Know More?
Interested in learning more about our solutions for the life science industry? Learn more about Kymanox at the 2023 PDA Universe of Pre-Filled Syringes and Injection Devices Conference, in Gothenburg, Sweden from October 17-18 2023, where the CEO and Founder will be giving the keynote address: The Inspiring Evolution of Parenteral Combination Products from 2004 to Today from an Entrepreneurial Perspective – And a Peek at What Is Coming.
References
- U.S. Food & Drug Administration, Inspection of Injectable Products for Visible Particulates, Draft Guidance for Industry, December 2021, Pharmaceutical Quality/CMC. https://www.fda.gov/regulatory-information/search-fda-guidance-documents/inspection-injectable-products-visible-particulates (accessed 2023-09-11)
- Knapp, J.Z. Origin, result and measurement of USP "essentially free" inspection for visible contaminating particles. PDA J Pharm Sci Technol. 2000, 54 (3), 218-232.
- Melchore, J. A. Sound Practices for Consistent Human Visual Inspection. AAPS PharmSciTech. 2011, 12(1), 215–221. DOI: 10.1208/s12249-010-9577-7
- Needle Gauge Chart. https://www.hamiltoncompany.com/laboratory-products/needles-knowledge/needle-gauge-chart (accessed 2023-09-11)
- Bukofzer, S.; Ayres, J.; Chavez, A.; Devera, M.; Miller, J.; Ross, D.; Shabushnig, J.; Vargo, S.; Watson, H.; Watson, R. Industry perspective on the medical risk of visible particles in injectable drug products. PDA J Parenter Sci Technol. 2015, 69(1), 123-139. DOI: 10.5731/pdajpst.2015.01037
- WHO. Best practices for injections and related procedures toolkit. 2010. https://www.who.int/publications/i/item/9789241599252
- Pountos, I.; Panteli, M.; Walters, G.; Bush, D.; Giannoudis, P. V. Safety of Epidural Corticosteroid Injections. Drugs in R&D. 2015, 16(1), 19–34. DOI: 10.1007/s40268-015-0119-3
- Benzon, H. T.; Chew, T.-L.; McCarthy, R. J.; Benzon, H. A.; Walega, D. R. Comparison of the Particle Sizes of Different Steroids and the Effect of Dilution. Anesthesiology. 2007, 106(2), 331–338. DOI: 10.1097/00000542-200702000-00022
- Langille, S. E. Particulate Matter in Injectable Drug Products. PDA J. Pharm. Sci. Tech. 2003, 67, 186–200. DOI: https://doi.org/10.5731/pdajpst.2013.00922
- Hill, S. E.; Heldman, L. S.; Goo, E. D. H.; Whippo, P. E.; Perkinson, J. C. Fatal Microvascular Pulmonary Emboli From Precipitation of a Total Nutrient Admixture Solution. Journal of Parenteral and Enteral Nutrition. 1996. 20(1), 81–87. DOI: 10.1177/014860719602000181
- Kinker JD, B. Intravitreal Silicone Oil Droplets after Multiple Avastin Injections. JOJ Ophthalmology. 2017, 2(3). DOI: 10.19080/JOJO.2017.02.555588
- Kahook, M. Y.; Liu, L.; Ruzycki, P.; Mandava, N.; Carpenter, J. F.; Petrash, J. M.; Ammar, D. A. High-molecular-weight aggregates in repackaged bevacizumab. Retina. 2010, 30(6), 887–892. DOI: 10.1097/IAE.0b013e3181d50cea
- Greenblatt, D. J.; Allen, M.D. Intramuscular Injection-Site Complications. JAMA: The Journal of the American Medical Association. 1978, 240(6), 542-544. DOI:10.1001/jama.1978.03290060044011
- Brierley, J. B. The penetration of particulate matter from the cerebrospinal fluid into the spinal ganglia, peripheral nerves, and perivascular spaces of the central nervous system. J Neurol Neurosurg Psychiatry, 1950, 13(3), 203-215. DOI: 10.1136/jnnp.13.3.203