Understanding Disinfectant Efficacy for Off-Label Microorganisms
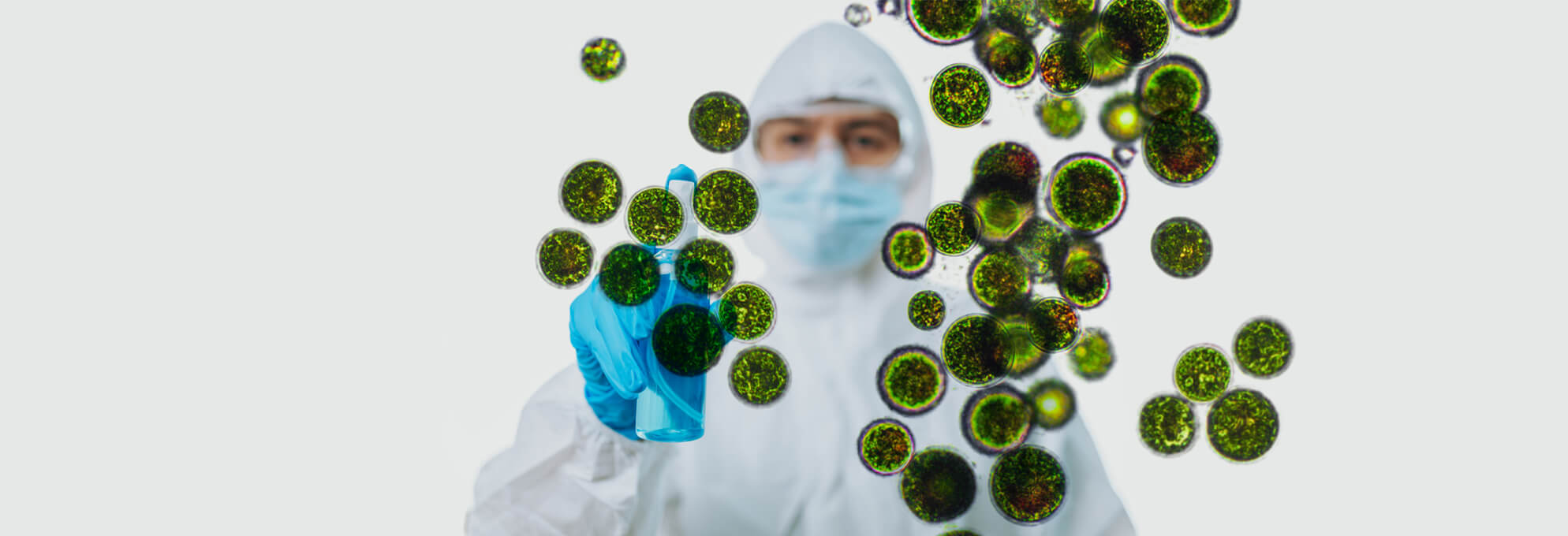
Environmental monitoring (EM) for microbial contamination in pharmaceutical and medical device cleanrooms is required and important in assessing the ongoing state of control. A hygiene program should focus primarily on reducing the ingression of microbes into controlled spaces from personnel, supplies and outside air. However, the appropriate use of disinfectants is also required to kill microorganisms that will trespass into the cleanroom and, potentially, into the product or preparation itself. Even then, viable bacteria and fungi will occasionally be recovered from EM and prompt an investigation.
While there are many potential root causes, the question below is frequently asked during the EM investigative process:
Our environmental monitoring recovered two bacteria, one identified as the Micrococcus/Kocuria species and the other identified as a Bacillus species. When I reviewed our disinfectants, I could not find these microorganisms listed on the EPA label or in our validation studies. Did we recover these microbes because our disinfectant is not effective against them?
For both types of microbes, the results of EM do not match any of the species listed on the labels for the disinfectants or isolates tested previously during the validation of the disinfectants. In either case, there is a concern that the disinfectants are ineffective in killing the isolate, which is understandable given some disinfectants can only kill certain microorganisms.
In addition, repeated application of antimicrobial agents is often associated with the perception that microbes will develop some level of resistance. Several arguments, however, support that a lack, or gap, of disinfectant efficacy is rarely the root cause for recovering microbes during the EM of cleanrooms. In order to help support these arguments, it is important to understand these three issues: (1) how disinfectants vs. antibiotics kill microbes, (2) the hierarchy of microbial susceptibility to disinfectants and (3) how disinfectants are regulated in the United States by the Environmental Protection Agency (EPA), in Europe and the United Kingdom under the Biocidal Products Regulation (BPR), and in GMP facilities as guided by USP <1072> Disinfectants and Antiseptics (1).
Mechanisms of Action of Disinfectants
Disinfectants kill microbes through various mechanisms that target components like the cell wall, cell membrane, proteins or nucleic acids. Disinfectants based on oxidizers, like hypochlorous acid, hydrogen peroxide or peracetic acid, readily react with multiple components of cells to cause irreversible damage leading to cell death. Non-oxidizing chemistries, such as quaternary ammonium or phenolic compounds, also cause lethal damage by denaturing and disrupting essential cellular structures and processes (1,2). In contrast, antibiotics and antifungal drugs inhibit the growth of or kill microbes by more specific mechanisms.
The nonspecific mechanism of action explains why disinfectants can kill a broad spectrum of microbes and why microbes are unlikely to develop resistance to disinfectants through repeated exposure, which is good news. However, the relatively nonspecific and reactive nature of disinfectants and sanitizers also explains why disinfectants can cause negative effects on organisms, such as humans, if not used as directed to reduce exposure. Additionally, disinfectants and sanitizers can react not only with macromolecules associated with living cells but also with dead cells, dirt and soiling. This issue is the basis for cleaning before disinfecting or using an EPA-registered one-step disinfectant that can disinfect in low-to-moderate soiling.
The Hierarchy of Susceptibility
While disinfectants function in nonspecific ways, differences among some types of microbes can impact the efficacy of disinfectants. This hierarchy of susceptibility is often described using a scheme originally proposed by Earle Spaulding regarding the use of disinfectants and sterilants on medical devices (3). (See Figure 1.)
All bacteria share some common properties because they are prokaryotic organisms. One of the most critical properties that impacts susceptibility is the lack of intracellular membranes. The vegetative form of a bacterial cell is essentially a balloon containing a mixture of proteins, carbohydrates and nucleic acids floating in what is called cytoplasm. Once a disinfectant penetrates the cell wall and membranes that make up the skin of the balloon, the chemicals can diffuse through the cytoplasm and interact with these intracellular targets.
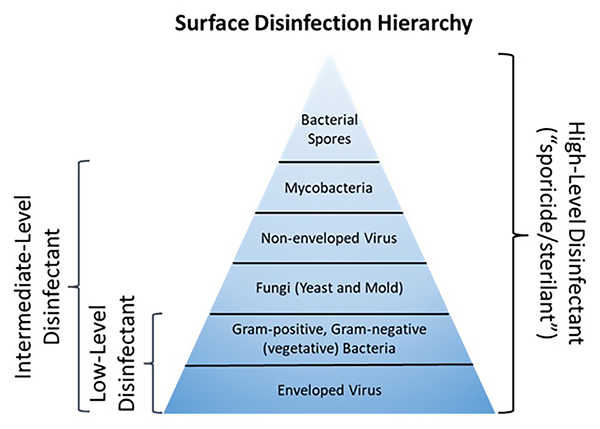
Some differences in the composition of the cell walls and membranes of bacteria can impact susceptibility, however. These differences in ultrastructure between Gram-negative and Gram-positive bacteria can be relatively subtle and non-impactful concerning most disinfectants. However, some bacteria (e.g., mycobacteria) or forms of bacteria (e.g., endospores) possess a unique cellular skin that greatly reduces the access of a surface disinfectant to intracellular targets. The intrinsic resistance of mycobacteria and bacterial spores to disinfectants warrants their location on the pyramid of susceptibility.
Unlike bacteria, fungi are eukaryotes like plants and animals. Nonetheless, the structure of fungal cells and their spores is considerably more diverse and complicated than vegetative bacteria, sometimes creating additional challenges of penetration and access of disinfectants to critical sites. There are five major phyla within the kingdom of fungi, with examples ranging from relatively simple cells like yeast to complex morphologies in true molds, that involve vegetative and reproductive components. While there is some debate among scientists and regulatory authorities regarding the term "sporicide" as related to bacterial vs. fungal spores, it is generally accepted that fungal spores are more susceptible to disinfectants than bacterial spores. The reduced susceptibility of eukaryotes to disinfectants also adds some margin of safety to reducing the deleterious toxicological effects of nontargeted organisms (plants, animals, humans) from unintended exposures to these chemical agents.
Although not typically the target of EM, the hierarchy of susceptibility of viruses is worth mentioning as the manufacturing of cell and gene therapy products (also known as advanced therapy medicinal products) continues to grow. The susceptibility of viruses to disinfectants is somewhat analogous to the availability of critical sites in bacteria and fungi. However, in the case of enveloped vs. non-enveloped viruses, the key to susceptibility is that a critical site associated with enveloped viruses is located on the outside of the skin (a proteinaceous shell called a capsid). While this relatively fragile, lipid-rich envelope is critical to evading host immune responses and infecting cells, it is highly susceptible to disruption by disinfectants. Despite the formidable challenges presented by enveloped viruses (e.g., SARS-CoV-2), once they enter the host, they are considered the easiest to deactivate by even low-level disinfectants.
Disinfectant Efficacy Testing in Support of Regulatory Approvals
Both EPA and BPR recognize the broad-spectrum activity of disinfectants. When products are registered as a specific type of disinfectant (e.g., bactericide, fungicide, virucide or sporicide), the EPA usually only requires testing against a few (or one) species of each type. (See Table 1.)
Table 1 EPA disinfectant claims summary. Information from EPA’s OCSPP 810 Guidelines (4).Claim Levels | Minimum Species for EPA Registration |
---|---|
Bacteria (broad-spectrum) | Staphylococcus aureus (Gram +) and (Salmonella enterica (Gram -) or Pseudomonas aeruginosa (Gram -)) |
Fungicide | Trichophyton interdigitale (athlete's foot fungus) |
Virucide | Claim the species (or surrogate) that is tested and approved |
Tuberculocide | Mycobacterium bovis (surrogate for M. tuberculosis) |
Sporicide | Endospores of Closteroides difficile (surface sporicide) or (Bacillus subtilis and Clostridium sporogenes (sterilant)) |
For example, to receive EPA registration as a fungicide, products must demonstrate an acceptable level of efficacy against Trichophyton interdigitale (athlete’s foot fungus) using a specified test method. Although other fungi can be tested with the same method, registration as a fungicidal disinfectant only requires testing against this species of fungi. If a registrant wants their product to carry a label claim against a different fungus, that species of fungus must also be tested.
Some ask, “Why are so few fungal species typically listed on the labels for fungicidal disinfectants?” Most products registered as disinfectants are targeted for use in healthcare or public-health settings, not cleanrooms. Therefore, testing focuses on the pathogens that cause the greatest concern for community- or healthcare-associated infections, not typical isolates from cleanrooms. While some fungi can be extremely pathogenic (e.g., Candida auris), most healthcare- and community-associated infections are caused by bacteria or viruses, respectively.
As shown in Table 1, the EPA regulatory scheme for claims against known viruses is more specific than that of bacteria and fungi. For example, while demonstrating efficacy against any virus might qualify a product to be a virucide, the EPA requires efficacy test data for each virus (or an acceptable surrogate) listed on the label. Yet, there are cases where the Agency has adopted a hierarchical argument that uses existing label claims to address emerging viral pathogens (5).
Thus, the regulatory approval process presents a mixed signal: Products can be broad-spectrum with testing against a few species is accepted, yet registered products cannot list specific species on their label unless they have been tested and authorities have approved the efficacy test results. A similar situation exists with disinfectants regulated under the BPR. To address these ambiguities, the EPA has explored the concept of susceptibility hierarchy and how it might be applied to the registration of disinfectants; however, this has not yet resulted in any changes in the registration process (6). Using a similar scheme for cleanroom facilities operating under GMP, USP <1072> Antiseptics and Disinfectants indicates testing only one to three species of bacteria, fungi and spores during the initial qualification of disinfectants (1). Subsequent efficacy testing is based on what types of microorganisms are typically isolated from the facility.
While registration with the EPA or BPR may not provide ultimate clarity regarding the spectrum of disinfectant activity, it is helpful to understand the conditions used in the efficacy test methods required for registration. The primary markets for registered disinfectants are healthcare and public facilities where microbial contamination is likely associated with fluids or particles from humans or other animals. As such, for disinfectants that do not require precleaning on the label instructions, products must be tested for efficacy in the presence of 5% bovine serum or comparable soiling simulants. As described above, disinfectants and sanitizers can become neutralized by interactions with soiling, so the conditions used in efficacy tests for registration are considerably harsher than those expected in a controlled environment such as a cleanroom.
Additionally, the bioburden used in efficacy tests for both registration and disinfectant validations is 104–106 microbial cells dried onto a relatively small area (3 cm2–25 cm2). Again, this is a greater challenge for the disinfectant than the level of contamination typically recovered in cleanrooms during EM of surfaces.
Summary
There are several issues to consider if an isolate is recovered during EM that is not listed explicitly on the EPA label or during previous disinfectant validations. Returning to the original question regarding Micrococcus/Kocuria or Bacillus species, the first description is complicated by the description of the isolate. A brief internet search or conversation with a microbiologist can help reveal that Micrococcus and Kocuria are two closely related genera of Gram-positive bacteria often associated with normal human skin. The two genera are so closely related phenotypically that many identification methods cannot differentiate between the two, so the lab indicates the result with both names. But to confirm if a disinfectant will kill these bacteria, the actual genus does not matter. Any product with broad-spectrum bactericidal claims used as directed is very likely to kill these bacteria. The likelihood increases if the disinfectant can also kill fungi, non-enveloped viruses or mycobacteria.
That these bacteria were isolated is not because the cells encountered the disinfectant and were unaffected, it was because the bacteria (likely shed from human skin or saliva) entered the cleanroom after the cleaning process or were not contacted by the disinfectant during the cleaning process. If these types of bacteria have been recovered repeatedly or the level of contamination exceeds action limits, appropriate responses would include a review or retraining of handwashing, gowning and disinfection procedures.
Regarding the Bacillus spp. isolate is a bit more complicated as the techniques used for EM do not differentiate between vegetative bacteria and spores. However, one should assume that recovery of a Bacillus species (or any of the dozens of related genera) originated from the spore form. Bacterial spores can enter the cleanroom from several environmental sources, including air, shoes and cardboard packaging. Interestingly, the one spore-former that can likely be ruled out is the species that appears on EPA-registered surface sporicidal agents, now identified as Clostridioides difficile (“C-diff”). Although these bacteria do produce bacterial spores and are a substantial healthcare concern, they are strictly anaerobic and cannot reproduce or produce spores outside the body of animals. Yet, it is the only spore-forming species for which EPA permits a surface-sporicidal (vs. sterilant/immersion) claim. Can a surface sporicidal disinfectant kill other genera and species of spore-forming bacteria as well as C-diff? The hierarchical scheme described above does not answer the question on its own. However, if one considers the bioburden (presence of soiling, number of spores, required log kill) associated with the C-diff test method, it is very likely that the sporicide will inactivate spore-forming bacteria recovered in cleanrooms. Rather than focusing on the disinfectant, reducing the ingress of the spores into the cleanroom is likely to yield improved outcomes.
References
- United States Pharmacopeial Convention, Inc. “USP <1072> Disinfectants and Antiseptics.” In USP–NF 2022, Issue 1. Rockville, MD, 2022.
- McDonnell, G. E. Antisepsis, Disinfection, and Sterilization: Types, Action, and Resistance; John Wiley & Sons, 2020.
- Spaulding, E. H. “Chemical Disinfection and Antisepsis in the Hospital.” J. Hosp. Res. 1957, 9, 5-31.
- U. S. Environmental Protection Agency. Product Performance Test Guidelines OCSPP 810.2200: Disinfectants for Use on Environmental Surfaces - Guidance for Efficacy Testing. EPA 712-C-17-004, Washington, DC, 2018.
- U. S. Environmental Protection Agency. Pesticide Registration: Emerging Viral Pathogen Guidance and Status for Antimicrobial Pesticides. EPA, Washington, DC, 2016. https://www.epa.gov/pesticide-registration/emerging-viral-pathogen-guidance-and-status-antimicrobial-pesticides
- U. S. Environmental Protection Agency. Ingredients Used in Pesticide Products: Final Summary of the Disinfection Hierarchy Workshop. EPA, Washington, DC, 2016. https://www.epa.gov/ingredients-used-pesticide-products/disinfection-hierarchy-stakeholder-workshop-documents